The VIU Molecular Modelling Research Group is working on the following projects.
Origin of Pressure Resistance in Proteins from Deep-Sea Organisms
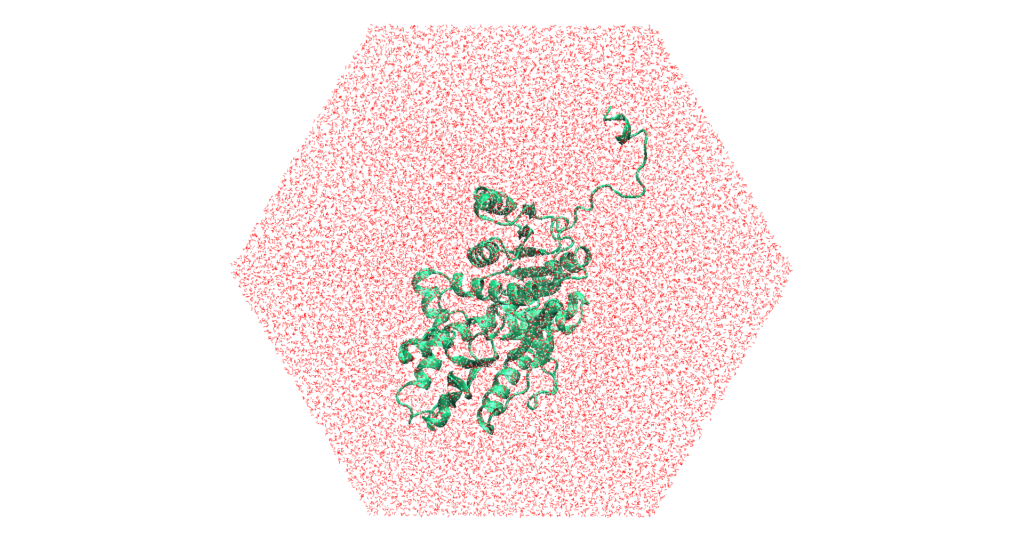
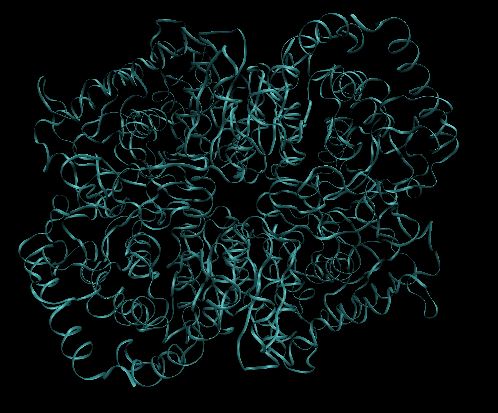
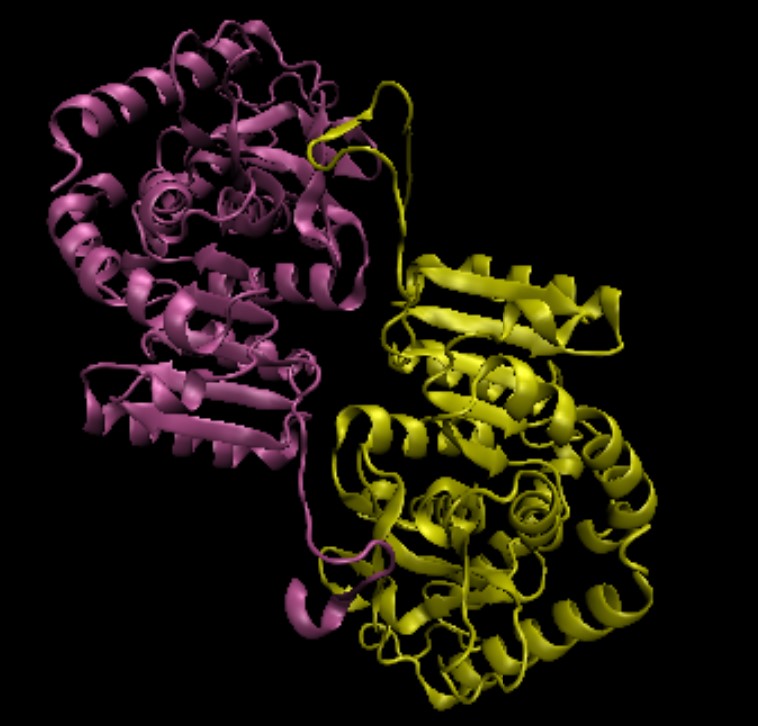
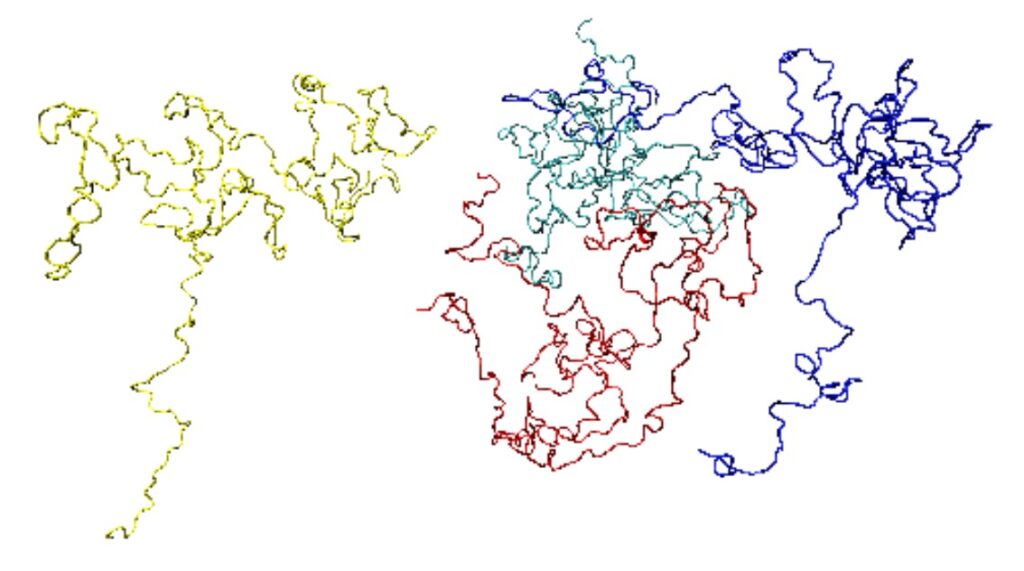
High pressure has serious effects on the macromolecules which make up living tissue. For example, increasing pressure will typically result in the dissociation of protein subunits, local conformational changes or in the most extreme cases, complete or partial protein unfolding. All of these changes will result in loss of protein function and are incompatible with life. Despite this, over 1500 species of marine organisms are estimated to live at ocean depths with pressures greater than 100 atmospheres. These organisms are able to survive thanks to evolutionary adaptations, such as amino acid substitutions, which allow them to cope with the stresses of high pressure. However, the mechanism by which these adaptations allow deep-sea proteins to resist pressure inactivation is currently unknown. We aim to answer this question by using computational techniques to study changes in the volumetric and thermodynamic properties of deep-sea and shallow-water proteins under pressure
Mechanistic Application of Volume Profiles
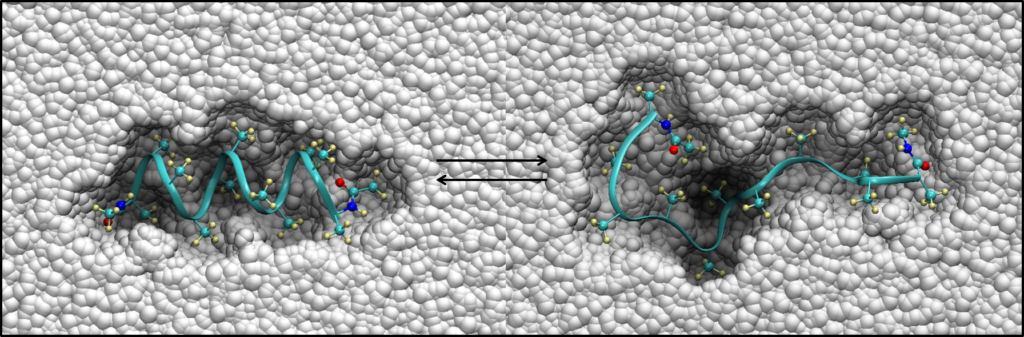
The effect of pressure on a reaction system is described by the activation and reaction volumes, defined as the pressure derivatives of rate constants and equilibrium constants respectively. These quantities provide information about the relationships between the partial molar volumes of the reactant, product and transition state (TS). However, mechanistic interpretation of these volumes has posed a challenge due to the lack of an accurate computational technique for relating the geometrical structure of a reaction system to its volume. We have formulated a theoretical methodology that can be used to describe and predict the effects of pressure on reaction systems using the concept of a volume profile. These profiles, which detail how the partial molar volume of a system changes over the course of a reaction, can be calculated using the recently developed Archimedean displacement model of molecular volume. This makes elucidation of TS structures possible, by comparing the theoretical volume profile with the experimentally-determined activation volume. This technique is especially useful for systems with a high degree of conformational flexibility whose TSs are not readily identified using standard computational methods, such as small proteins and peptides
Biological Activity of Carfentanil Precursors
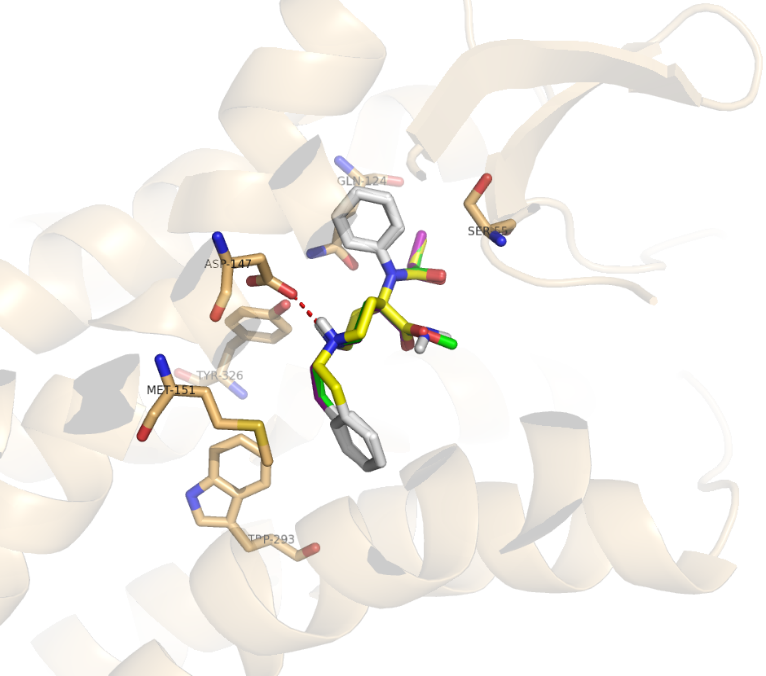
Unintentional drug overdose is the leading cause of unnatural death in BC, with the majority of those deaths due to synthetic opioids like fentanyl and carfentanil. Drug checking services using paper-spray mass spectrometry can detect trace amounts of carfentanil in illicit drug samples. Recent analysis in Victoria, BC found that illicit drug samples containing carfentanil also contained other molecules which were structurally similar to carfentanil. These molecules were proposed to be synthetic precursors to carfentanil. It is unknown whether these precursor molecules are biologically active and if so, whether they are also toxic. We are using molecular modeling to study the interaction between these precursor molecules to the ยต-opioid receptor, which is the protein responsible for initiating the cell-signaling pathway that results in the toxic side effects of opioids
Pressure-Driven Molecular Machines
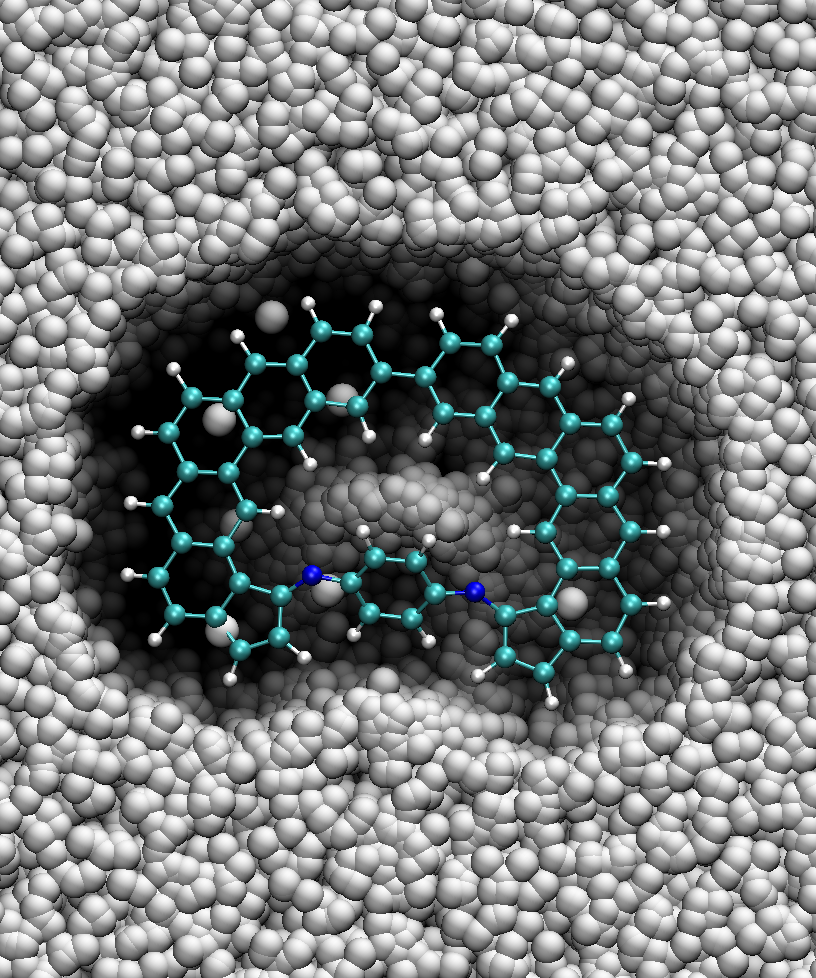
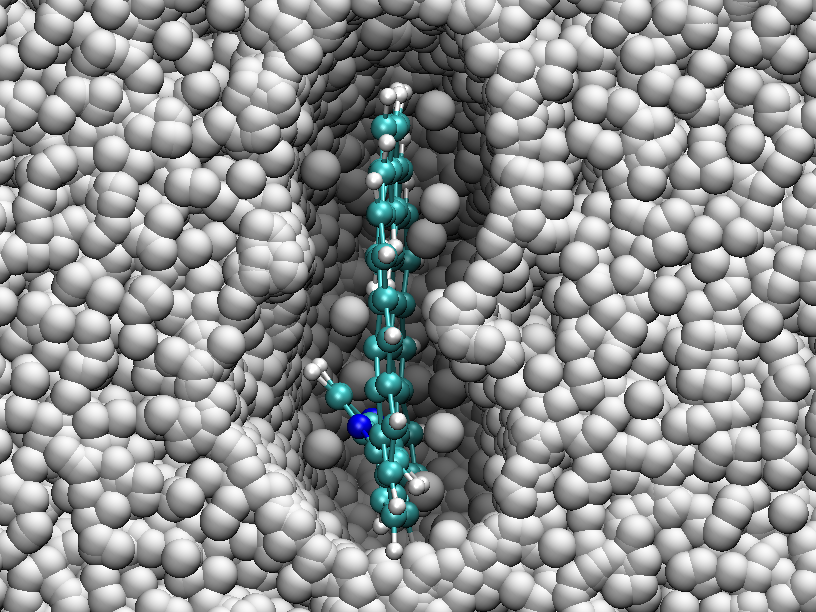
Molecular machines are a class of molecules that perform an output function for a given input stimulus. These machines can be biological in origin, such as enzymes, ribosomes or motor proteins, or they can be synthetically designed. Recent years have seen the development of a wide variety of synthetic molecular machines including molecular switches, wires and rotors for potential use in nanodevices. The output function of a molecular machine is accomplished through a conformational change, which results in a useful change in the properties of the molecule. Since different conformations of molecules are likely to have different volumes, pressure is an ideal tool to drive these conformational changes. We use density functional theory and molecular dynamics simulations to design pressure-controlled molecular machines, including a unidirectional rotor and molecular wires.